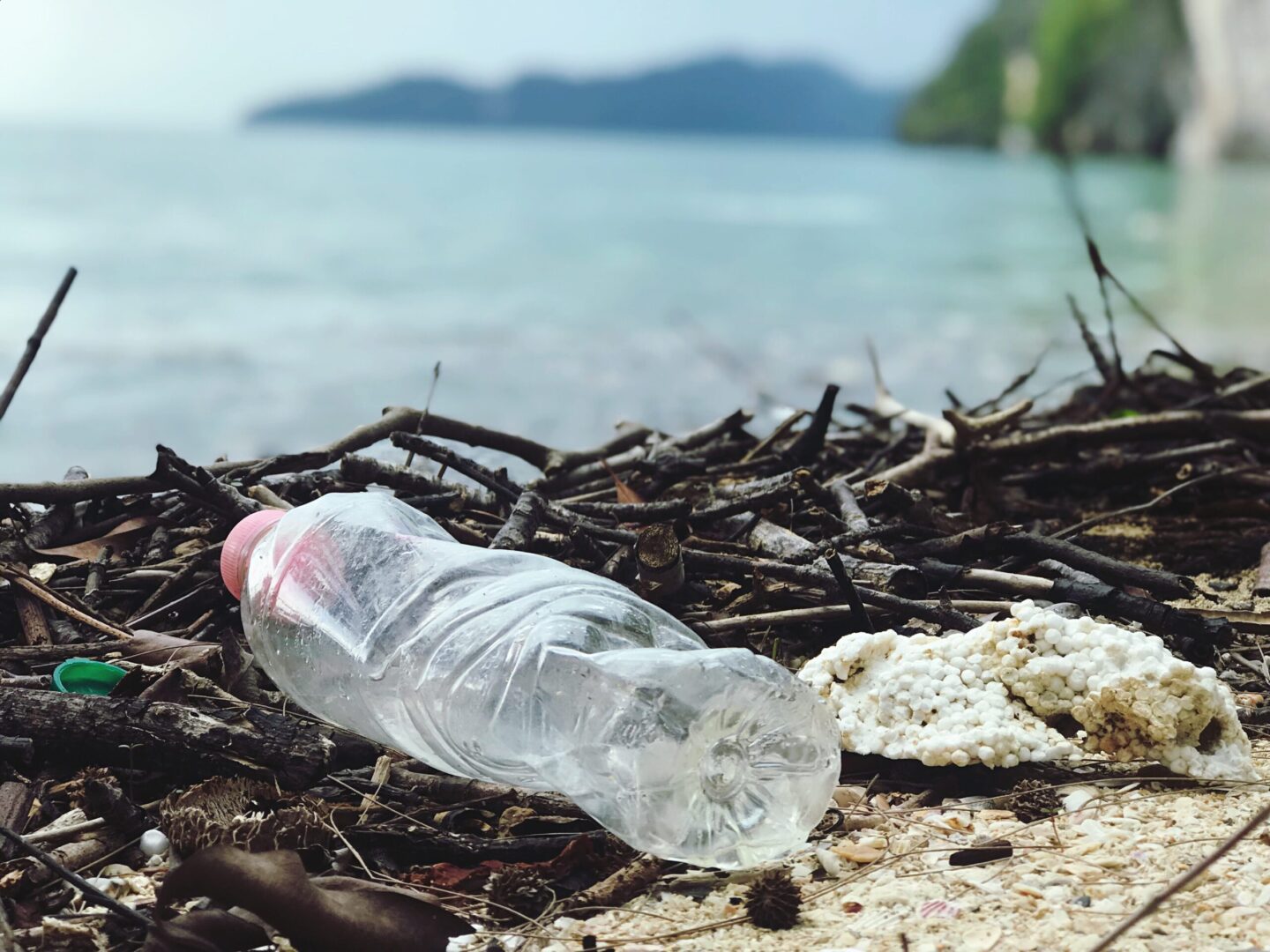
Is upcycling plastic waste using light an option? As a society, we have made a lot of plastic, (more than 8 billion tons historically) and we struggle with any sort of reasonable solution of how to get rid of it. Most ends up in landfills or as litter or we dump it in the ocean. This plastic waste breaks down to microplastics polluting our soil and water and poisoning wildlife. Some of our plastic is burned for energy and turned into CO2 adding to our excesses. And occasionally some of it ends up getting recycled and reused, albeit for lower value materials that themselves likely end up in a landfill. All of it is highly energy intensive. Of all the oil that we dig out of the ground about 6 percent simply just goes to making more plastic junk.
We have so much plastic that that no single solution or application will likely ever solve all our plastic waste problems. On the bright side, all of this plastic waste represents a vast potential feedstock for the chemical industry (we’re liberally using the term “bright side” here). A recent review on plastic waste valorization methods describes recent numerous advances in thermocatalysis, electrocatalysis, biocatalysis and photocatalysis (Ref 1). Often these methods require high temperature and pressure, (read: energy), precious metal catalysts and generate a significant amount of CO2. To be economically viable, upcycling of plastic needs to take low-cost plastic waste and turn it into something of higher value. Three polymers, polyethylene (PE), polypropylene (PP) and polystyrene (PS) represent more than 60% of all plastic waste. We don’t often think about these inert polymers with strong non-polar C-C bonds as useful starting materials but therein lies the challenge.
A recent paper by Reisner and coworkers at the University of Cambridge looks to tackle this problem using visible-light photocatalysis (Ref 2). The authors set out to recover benzoic acid from the deconstruction of polystyrene polymers with a visible light catalyzed hydrogen atom transfer catalysis (HAT) and a blue LED (Figure 1). In a model system, the authors use polystyrene with a weight-average molecular weight (Mw) of ~260,000 dissolved in ethyl acetate with 0.2 equiv. fluorenone as the photocatalyst along with 1 equiv. of H2SO4. The reaction is irradiated at room temperature with a 14 W 450 nm LED with O2 added at ambient pressure. The temperature of the reaction increased to 50 °C.
Figure 1: Visible-light HAT catalyzed decomposition of polystyreneThe reaction was then monitored in multiple ways. Product yields for the aromatic products were determined by HPLC (UV-vis and mass spec) and ¹H NMR while the average Mw of the reaction mixture was determined by gel permeation chromatography (GPC). GC-MS was used to detect volatile products. After 16 hours, the major product in the mixture was benzoic acid with a yield of 30% (38% at 48 h). No PS peak was observed by GPC after 16 h. Ethyl benzoate, (10%) acetophenone (6%), and benzaldehyde (1%) were also detected by ¹H NMR along with phenylglyoxylic acid. At 48 h, the overall yield of aromatic monomers was around 60% with the remaining mass balance accounted for by functionalized oligomers ranging in mass from 200-800. In the headspace of the reaction, 21% CO and 7.5% CO2 were detected. At the completion of the reaction 95% of the fluorenone catalyst remained and was able to be recycled. As for controls, no reaction was observed in the absence of the acid, photocatalyst or light (temperature at 50 °C). A control without PS showed that none of the aromatic products observed derived from the fluorenone catalyst. The authors also looked at a variety of organic catalysts and conditions. The selected reaction was then utilized on gram scale using pure polystyrene and real-world packing foam. Here, isolated yields of ~38% at gram scale demonstrate the practical and scalability of the reaction.
As for the proposed mechanism (Figure 2A), an excited state of the photocatalyst generates an organic radical on the tertiary carbon of the polystyrene. This radical can react with O2 to generate peroxyl radical which performs a second hydrogen atom abstraction (B). The peroxide intermediate requires 1 equivalent of acid (C) in order to lose water and subsequently undergoes a β-scission to break the carbon-carbon chain (D), generating a carbon centered radical and C=O bond. O2 trapping of the radical and repeated oxidations generate the phenylglycoxylic acid (E) which decarboxylates to generate benzoic acid (F). Extensive DFT experiments and radical trapping experiments with TEMPO support the mechanism.
Figure 2: HAT mechanism for the deconstruction of polystyrene
Overall, the authors demonstrate a polystyrene deconstruction reaction via C-H oxidations that operates at ambient temperature and pressure with a re-usuable organic photocatalyst under visible light. The process is scalable and produces benzoic acid from real-world plastic waste. We look forward to the authors approaches to tackle additional polymers such as polypropylene, polyethylene and polyvinyl chloride.
- Zhou, H.; Wang, Y.; Ren, Y.; Li, Z.; Kong, X.; Shao, M.; Duan, H. Plastic Waste Valorization by Leveraging Multidisciplinary Catalytic Technologies. ACS Catal. 2022, 12, 9307–9324. https://doi.org/10.1021/acscatal.2c02775.
- Li, T.; Vijeta, A.; Casadevall, C.; Gentleman, A.; Euser, T.; Reisner, E. Bridging Plastic Recycling and Organic Catalysis: Photocatalytic Conversion of Polystyrene into Benzoic Acid via C-H Oxidation Pathway. ACS Catal. 2022, 12, 8155–8163. https://doi.org/10.1021/acscatal.2c02292.